Magnetic Resonance Imaging
We explore MRI physics, signal formulation, image reconstruction, and tissue modeling. Our goal is to develop innovative MRI methods for understanding fundamental pathophysiological processes of diseases and providing tools to assess tissue biological structures and activities in the brain, joints, and body.
A full list of our publications in this category can be found here.
Research Highlight
Quantitative MRI for Tissue Characterization
MRI is a versatile and powerful imaging method used in clinical diagnosis and scientific research. It provides detailed soft-tissue images and various contrast options without using radiation. This enables the capture of functional, hemodynamic, metabolic, and high-resolution anatomical images for a comprehensive examination. MRI also allows for the quantitative measurement of tissue properties in living organisms. There is a growing interest in using quantitative MRI for routine clinical assessments as it offers increased sensitivity to different diseases and may facilitate early identification of pathologies. Additionally, quantitative measurements can provide valuable information about tissue composition and microstructure. Our research group focuses on quantitative MRI, particularly in the areas of multi-contrast spin-lattice relaxation time (T1), spin-spin relaxation time (T2), spin-lattice relaxation time in the rotating frame (T1ρ), magnetization transfer imaging, ultra-short echo time imaging, and susceptibility imaging in the brain, body, and musculoskeletal system applications.
T1, T2, and T1ρ Relaxation Mapping
The T2 and T1ρ relaxation parameters are extensively studied for assessing tissue degeneration in knee and brain diseases. T2 relaxation time is the most commonly used method and has the most extensive body of literature. It can detect subtle changes in the macromolecular and water content as well as the ultrastructure of tissue extracellular matrix, which are associated with early tissue degeneration. These changes occur before the onset of visible tissue damage. Cartilage T1ρ can indicate changes in the cartilage extracellular matrix with high sensitivity to the loss of important macromolecules, making it a potential tool for early detection of Osteoarthritis. T1 time is also actively researched and has been found to correlate with mechanical property changes of cartilage, different from T2 and T1ρ. It also shows high sensitivity to brain tissue degeneration.
We have developed imaging techniques to thoroughly assess tissue microstructure and composition using advanced quantitative MR relaxometry. Our MR imaging protocol involves performing bi-component T2 mapping of cartilage and brain using two steady-state imaging sequences. We acquire Spoiled gradient-echo (SPGR) scans over multiple flip angles to infer T1 information, and Balanced steady-state free precession (bSSFP) scans over multiple flip angles to infer combined T2/T1 information. These SPGR and bSSFP images are input into a bi-component tissue model to decouple two water pools. When evaluating cartilage matrix, bi-component T2 maps can be used to assess proteoglycan and collagen content. In brain tissue assessment, this technique can evaluate myelin integrity through myelin water characterization.
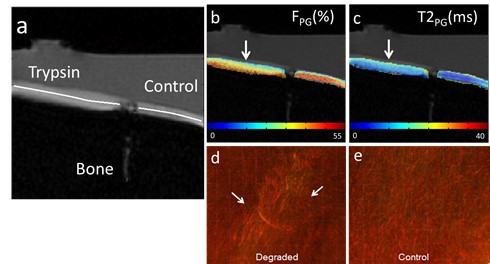
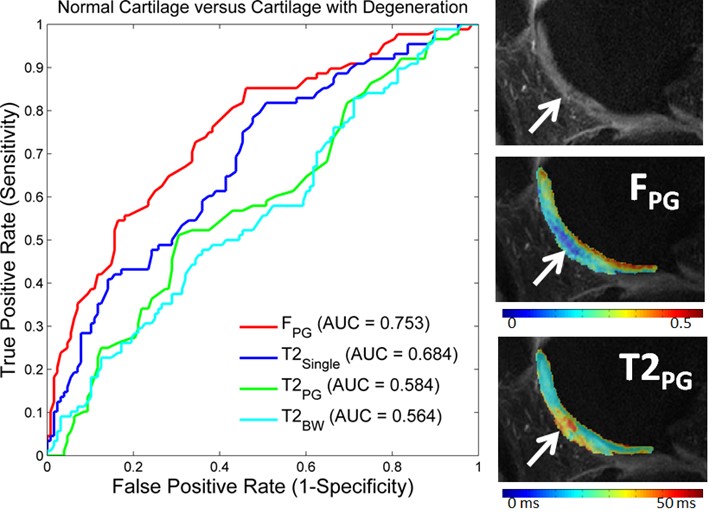
- Liu F, Chaudhary R, Hurley SA, Munoz del Rio A, Alexander A, Samsonov A, Block WF, Kijowski R: Rapid Multi-Component T2 Analysis of the Articular Cartilage of the Knee Joint. J Magn Reson Imaging. 2014; 39(5):1191-7.
- Liu F, Choi K, Samsonov A, Spencer R, Wilson J, Block W, Kijowski R: Articular Cartilage of the Human Knee Joint: In Vivo Multicomponent T2 Analysis at 3.0 T. Radiology. 2015; 277(2): 477-88.
- Liu F, Samsonov A, Wilson J, Blankenbaker D, Block W, Kijowski R: Rapid In Vivo Multi-Component T2 Mapping of Human Knee Menisci. J Magn Reson Imaging. 2015; 42(5): 1321-8.
- Liu F, Chaudhary R, Samsonov A, Block WF, Kijowski R: Multicomponent T2 analysis of articular cartilage with synovial fluid partial volume correction. J Magn Reson Imaging, 2016; 43(5): 1140-7.
Magnetization Transfer Mapping
Cross-relaxation imaging is a promising technique that can investigate the tissue extracellular matrix by utilizing the magnetization transfer (MT) effect between the water protons and the macromolecular protons of the tissue. This imaging method can offer detailed measurements of the MT parameters at the voxel level, including the fraction of the macromolecular bound protons (f) and the T2 relaxation time of macromolecular bound protons. Our studies have demonstrated the sensitivity of MT parameters to changes in the cartilage extracellular matrix through both ex-vivo and in-vivo research. Additionally, we have successfully imaged the whole brain’s multi-component T2 and MT parameters using an efficient imaging acquisition protocol. The MT parameters obtained through cross-relaxation imaging may provide valuable insights into the collagen fiber network of cartilage, the myelin content of brain tissue, and various macromolecule content in other parts of the body. By combining advanced relaxation imaging and MT imaging, we can develop sensitive and specific imaging biomarkers to comprehensively assess the contents of macromolecules in disease formation and progression.
Ongoing development in the lab aims to acquire multiple contrast MT and relaxation parameters simultaneously. This is achieved through an integrated acquisition framework that compensates for system imperfections and motion, while maintaining a super high spatial resolution, low signal-to-noise ratio, and short scan time.
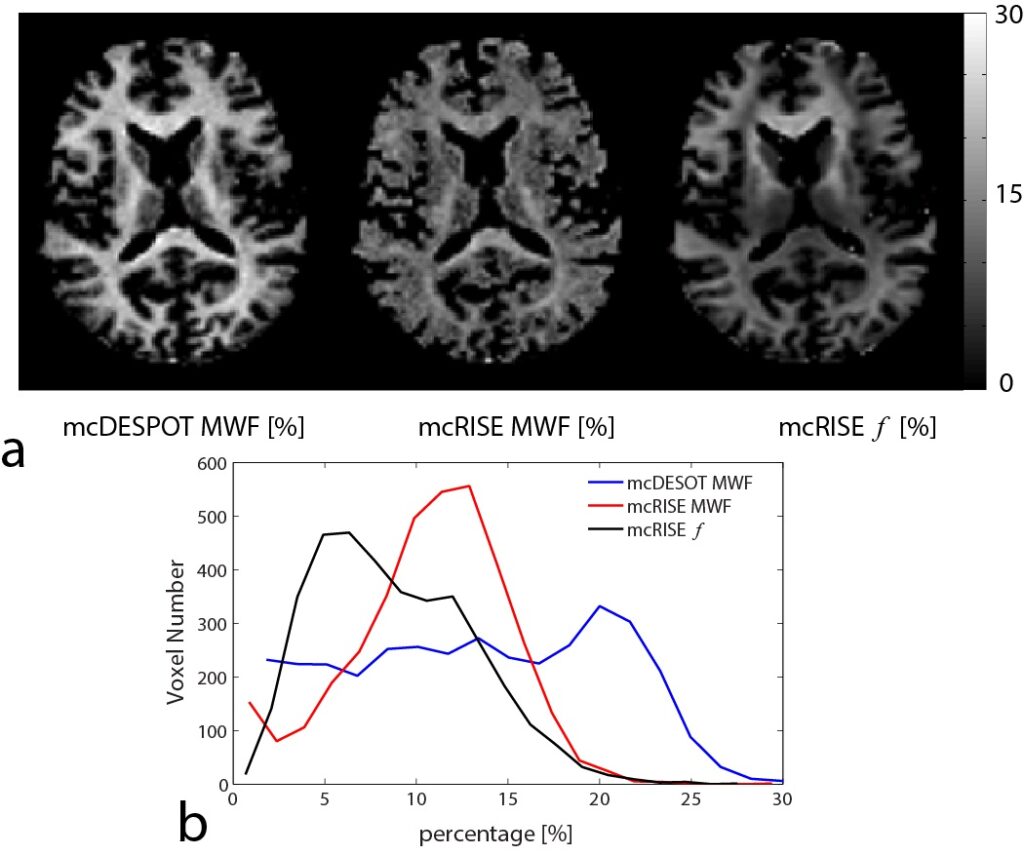
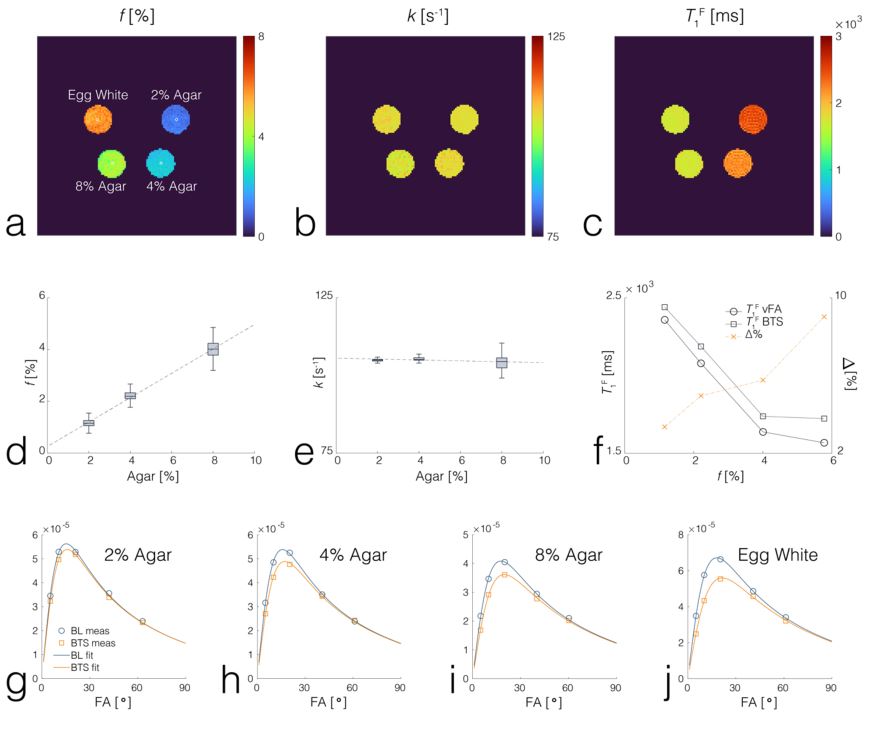
- Liu F, Block W, Kijowski R, Samsonov A: Rapid Multi-Component Relaxometry in Steady State with Correction of Magnetization Transfer Effects. Magn Reson Med. 2016; 75(4): 1423-33.
- Liu F, Velikina JV, Block W, Kijowski R, Samsonov A: Fast Realistic MRI Simulations Based on Generalized Multi-Pool Exchange Tissue Model. IEEE Trans Med Imaging. 2017; 36:527-37.
- Jang A, Han P, Ma C, El Fakhri G, Wang N, Samsonov A, Liu F: B1 Inhomogeneity-corrected T1 Mapping and Quantitative Magnetization Transfer Imaging via Simultaneously Estimating Bloch-Siegert Shift and Magnetization Transfer Effects. Magn Reson Med. 2023; 90(5): 1859-1873.
Ultra-short Echo Time Imaging
Musculoskeletal tissues like tendons, ligaments, and meniscus contain a lot of organized collagen fibers, which make them appear dark in regular MRI scans because their signal decays very quickly. Recently, ultrashort echo time (UTE) techniques have been developed to capture this rapid signal decay in these tissues. By using multiple very short echoes (as short as 0.008 msec), these techniques can calculate the ultra-short echo time T2* (UTE-T2*) relaxation time of tendons, ligaments, meniscus, and cortical bone. We have created new multi-component UTE-T2* acquisition and image processing methods to improve the imaging ability of UTE for better understanding tissue properties despite low signal and various system confounding factors.
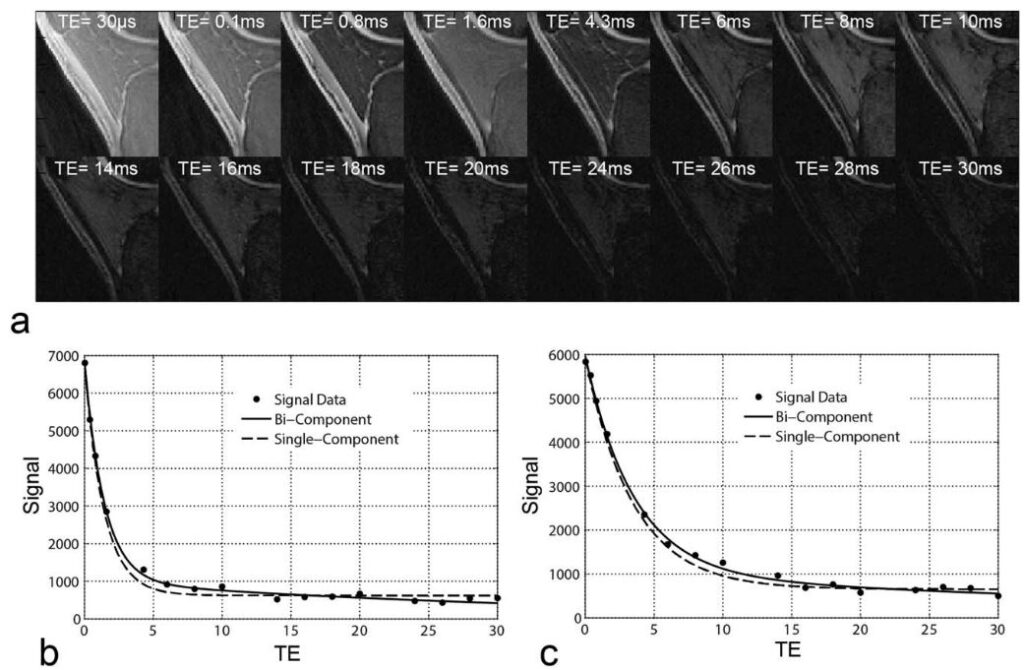
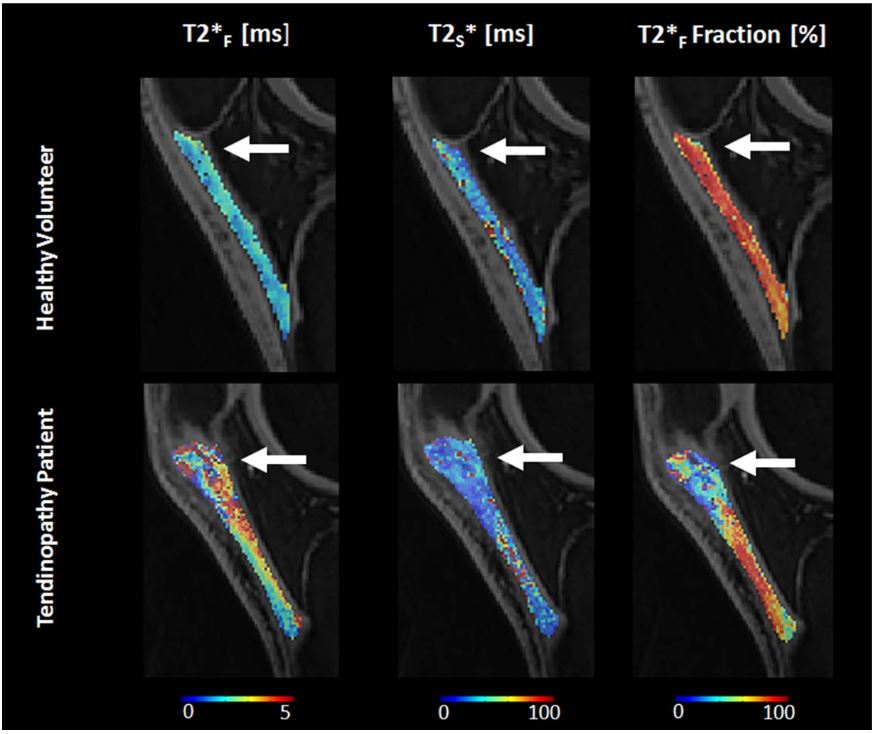
- Kijowski R, Wilson J, Liu F: Bi-Component Ultra-Short Echo Time T2* Analysis for Assessment of Patients with Patellar Tendinopathy. J Magn Reson Imaging. 2017; 46 (5), 1441-1447.
- Liu F, Kijowski R: Assessment of Different Fitting Methods for In-Vivo Bi-Component T2* Analysis of Human Patellar Tendon at 3.0T. Muscle, Ligaments and Tendons Journal. 2017; 7(1):163-172.
- Loegering I, Denning S, Johnson K, Liu F, Lee K, Thelen D: Ultrashort echo time (UTE) imaging reveals a shift in bound water that is sensitive to sub-clinical tendinopathy in older adults. Skeletal Radiology. 2021; 50 (1), 107-113.
Rapid MRI using Compressed Sensing, Parallel Imaging and Non-Cartesian Acquisition
We are researching different techniques to speed up MRI scans, such as compressed sensing, parallel imaging, and a combination of both. Additionally, we are developing new MRI sequences to improve non-Cartesian k-space sampling, aiming for efficient scanning, fewer imaging artifacts, three-dimensional volumetric imaging, and better reconstruction for brain, knee, and body imaging.
Rapid 3D Knee MRI using Compressed Sensing
Commercially available on most MRI vendor platforms, three-dimensional fast spin-echo (3D-FSE-CUBE) sequences can acquire thin continuous slices through joints. These slices can be reformatted in any orientation, eliminating the need to repeat sequences with identical tissue contrast in multiple planes. The use of 3D-FSE-CUBE sequences in clinical practice could significantly decrease MRI examination times, improving patient comfort and increasing the clinical efficiency of the MRI scanner.
Currently, 3D-FSE-CUBE sequences have long scan times to achieve high isotropic resolution. Compressed sensing (CS) is a method that could reduce the scan time by acquiring less image data through k-space undersampling. We conducted studies to explore the possibility of using CS to speed up 3D-FSE-CUBE imaging of the knee and to determine the best imaging parameters of CS for improved image quality in assessing various joint structures.


Novel Non-Cartesian MRI
Our group is working closely with Dr. Li Feng from New York University to develop, optimize, and evaluate non-Cartesian imaging in various clinical applications. We are particularly interested in the GRASP imaging technique, originally developed by Dr. Feng and his colleagues at New York University. We are exploring the integration of GRASP and its variants with our AI-based reconstruction techniques, including SANTIS, MANTIS, and RELAX, to achieve faster image generation, improved quality, accurate quantification, and enhanced spatial-temporal resolution while ensuring algorithm convergence.
The GRASP project represents a decade of innovation by our team consisting of imaging scientists, clinicians, and our industry partners. The GRASP paper was announced as the third most-cited MRM paper at the 2017 ISMRM annual meeting, and the XD-GRASP paper was announced as the top most-cited MRM paper at the 2019 ISMRM annual meeting. With the rise of Artificial Intelligence in recent years, we are now aiming to integrate GRASP MRI with deep learning approaches to enable further improvement in reconstruction quality and speed, as well as new uses of this imaging framework. The initial feasibility of deep-learning-enabled golden-angle radial MRI has been demonstrated by us with a technique called SANTIS (see Deep Learning for Rapid MRI), and we are in the process of developing new quantitative imaging methods based on a combination of GRASP with deep learning.
by Dr. Li Feng
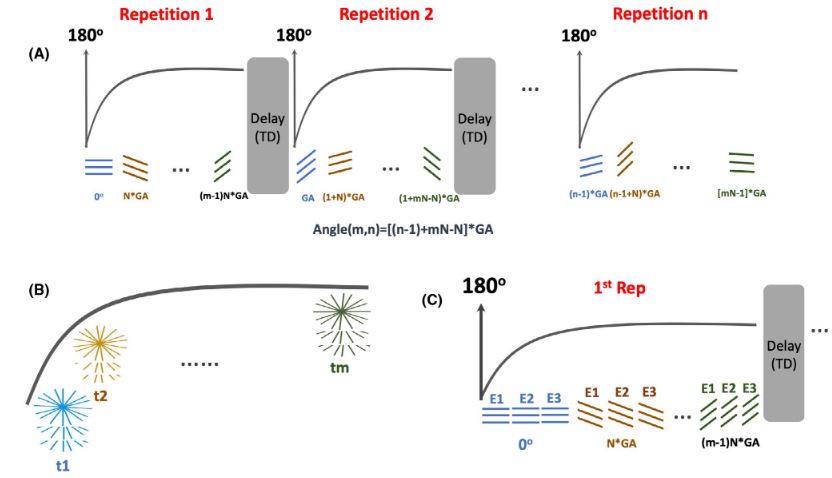
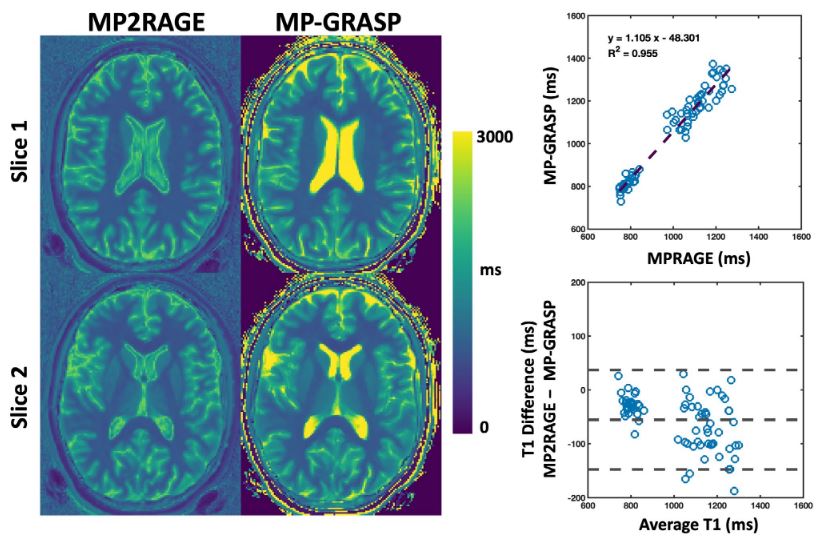
- Feng L, Wen Q, Huang C, Tong A, Liu F, Chandarana H: GRASP-Pro: imProving GRASP DCE-MRI through self-calibrating subspace-constraint and automated selection of contrast phases. Magn Reson Med. 2019; 83 (1), 94-108
- Feng L, Liu F, Soultanidis G, Liu C, Benkert T, Block K, Fayad Z, Yang Y: Magnetization-prepared GRASP MRI for rapid 3D T1 mapping and fat/water-separated T1 mapping. Magn Reson Med. 2021; 86 (1), 97-114.